To drive down the cost of electrolyzers, production would need to ramp up. Current manufacturing is on the scale of megawatts (MW), but production on the scale of gigawatts (GW) would be needed to drive overall prices down into the $200-300/kW range. Like other technologies such as wind, solar, and ethanol, capital costs are high in this early phase of commercialization. As manufacturing ramps up, costs are expected to fall.
As will be seen in this section, despite green hydrogen and green ammonia’s application potential in Minnesota and the Midwest, the current policy framework may not be sufficient for developing the industry here. Existing low carbon fuel credit standards on the West Coast are designed to support and protect their West Coast market: wind or solar process energy that makes clean fuels in the Midwest will not deliver carbon credits under the West Coast regulatory structures. Another option, the federal tax credit for carbon credit and sequestration technologies, may present the possibility of additional incentives for green ammonia in the future, though it is unclear at this point whether green ammonia/urea would qualify for the credit. A third possibility—a green hydrogen tax credit—has been proposed but not yet passed. After consideration of these existing structures, the final part of this section will explore factors to consider with respect to a potential Midwest low carbon fuel standard—particularly the choice between physical and virtual power purchase agreements.
Existing Low Carbon Fuel Standards (LCFS)
Marketplaces providing bankable financial incentives for clean fuels (like green hydrogen and ammonia) exist only on the West Coast: California, Oregon, Washington and British Columbia.51 These states’ Low Carbon Fuel Standards (LCFS) rely on annual carbon intensity (CI) benchmarks,52 defined as the quantity of lifecycle greenhouse gas emissions per unit of fuel energy.53
Compliance with the LCFS relies on a carbon credit and deficit accounting system which is expressed in metric tons of CO2e. The carbon credits are monetized when regulated parties (businesses that produce or sell fossil fuels like gasoline, diesel, propane, and natural gas in these states)54 must purchase credits in a credit banking system to offset their carbon deficits. Regulated entities accumulate deficits when they produce fuels with a higher carbon intensity score than the LCFS target.55
For no- or low-carbon fuel project developers, there are three primary methods of generating carbon credits for incentive payments under LCFS standards:
- Fuels-based crediting: Entities that produce zero or low carbon fuels like green hydrogen, generate credits based on emissions reduced in comparison to the LCFS carbon intensity baseline. These credits incentivize project developers to produce clean fuel options for the LCFS West Coast markets.56
- Project-based crediting: These kinds of projects reduce emissions across the petroleum supply chain, including carbon capture and sequestration.57
- Zero-emissions vehicle infrastructure crediting: Installation of hydrogen fueling and DC electric fast charging infrastructure generates carbon credits based on capacity. These credits assist medium and heavy duty zero emissions vehicles in particular.58
To qualify as a low-carbon process energy source for the production of a low-carbon fuel in the West Coast LCFS markets, the energy from the generation source must be directly consumed in the fuel production process according to specific parameters:
- The low-carbon intensity electricity must be supplied from generation equipment under the control of the low-carbon fuels producer. Any renewable energy certificates associated with the electricity cannot be retired or claimed under any other program with the exception of the federal Renewable Fuel Standard.
- The generation equipment is directly connected through a dedicated line to the fuels production facility such that the generation and load are both physically located on the customer side of the utility meter.
- The low-carbon fuel facility’s electric load is sufficient to match the amount of low-carbon intensity electricity claimed using a monthly balancing period.59
This fuel-based crediting method does not allow indirect accounting mechanisms for low-carbon process energy, such as the purchase of renewable energy certificates or “greensleeve” power purchase agreements from renewable generation resources, unless those facilities supply power to one of the West Coast’s electric grid balancing authorities.60 To qualify, the wind or solar electric facility providing the process energy for low-carbon fuels production must be “behind the meter,” or feed renewable electricity directly into the grid under the control of West Coast states for the process energy to earn carbon credits.
These strict parameters put Minnesota and Midwestern-made green hydrogen, green ammonia, and biofuels at a competitive disadvantage in the West Coast markets, since any wind or solar process energy that makes clean fuels will not deliver carbon credits under the West Coast LCFS regulatory structures. Several non-West Coast states challenged the parameters of West Coast LCFS markets on legal grounds they violate the federal government’s exclusive authority to regulate commerce amongst the states. The Ninth Circuit Court of Appeals, however, denied these challenges, ruling that these states were merely exercising their state “police” powers by regulating in-state parties and in-state transactions via a carbon lifecycle accounting method for transportation fuels.61
45Q: Federal Tax Credit
The federal tax credit for carbon credit and sequestration technologies62 (also known as “45Q tax credit”) may present the possibility of additional financing options for green ammonia in the future. Recently, the Internal Revenue Service (IRS) set forth regulatory requirements for demonstrating the “secure geological storage of carbon oxide” and places heavy emphasis on permanent geological storage.63 However, the 45Q tax credit does include “chemical conversion of such qualified carbon oxide to a material or chemical compound in which such qualified carbon oxide is securely stored.”64 The IRS declined to comment on whether specific chemical processes that include fixing carbon dioxide into specific compounds would qualify for the 45Q tax credit.65 As it stands, the IRS has not made clear whether sequestering carbon dioxide in the form of green urea, and ultimately plant material, would qualify (and how) for the 45Q tax credit.
H2 Tax Credit
Proposed in 2021, the Build Back Better Act would have provided incentives to stimulate production of clean hydrogen. Producers could have utilized either a production tax credit of up to $3 a kilogram for 10 years or an investment tax credit of up to 30% of the cost of the electrolyzer and other equipment, claimed in the year the equipment was put into service.66 The actual credit amount per kg of hydrogen would have varied based on the quantity of CO2 emitted to produce a kilogram of hydrogen, as measured on a lifecycle basis.67 In July 2022, the US Inflation Reduction Act also included an up to $3 per kilogram tax credit for low-carbon hydrogen. Exact amounts of the credit are to be determined by the hydrogen’s full lifecycle greenhouse gas emissions and a project’s ability to meet “multiplier” criteria for in services dates and wage and labor requirements.68
Impacts of Potential Federal Incentives
Table 8 highlights the potential impact that the 45Q tax credit and green hydrogen tax credit could play in scaling commercial investment in a green hydrogen industry. The values of the credits shown below are conservative estimates of the potential tax credit values. The 45Q tax credit value is estimated to provide a payment of $35/ton of CO2 avoided while the H2 tax credit is estimated at $1.50/kg H2.
The unlevered internal rate of return (IRR) of a 100 tpd plant, without any tax credits or incentives, would not cash flow today at $600/tons. However, as Table 8 shows, a $1.50/kg H2 tax credit could, under existing conditions, increase the unlevered internal rate of return into positive percentages. Table 8 further shows that with either of these incentives facilities could capture positive IRRs with either a decrease in the cost of cells or an increase in the utilization factor, alone. With both increasing utilization factor and a decline in the cost of cells, these incentives would drive facilities into territory that would attract commercial investment.
Table 8: Unlevered IRR Impact of Potential Federal Incentives

Getting Local: Minnesota Department of Agriculture’s Renewable Chemical Production Incentive Program
As mentioned previously, Minnesota farmers purchase approximately 350,000 tons of urea for application to farm fields.69 State law already exempts from sales and use tax the purchase or use of chemical fertilizers for use or consumption in agricultural production.70 In order to incentivize green urea purchase and use amongst farmers, policymakers could establish farm income tax credits or subsidize green urea production through mechanisms like the Minnesota Department of Agriculture’s Renewable Chemical Production Incentive Program. This program already bases incentives on pounds produced in a fiscal quarter, though it is geared toward cellulosic biomass and sugars.71 There is a strong market for urea sales and use, and further economic study would assist in identifying effective ways to finance these developments.
Getting Local: Frameworks for a Midwest Low-Carbon Fuel Standard
Thoughtful design of a Midwest low-carbon fuel standard could attract investors to Minnesota and help this budding industry weather market uncertainties. Minnesota’s low-cost wind generation positions it well to use clean energy to produce green ammonia for fertilizer or fuel. Most, if not all, utility-scale wind and solar generation facilities in Minnesota participate in the renewable energy tracking platform, M-RETs72, and feed electricity into the Midcontinent Independent System Operator (MISO), the grid balancing authority for 15 states and the province of Manitoba, Canada.73
Physical delivery power purchase agreements (PPAs) and virtual power purchase agreements (VPPAs) of green energy may be the simplest paths for qualifying green ammonia under an environmental attribute market program such as a low carbon fuel standard.
Physical PPA Framework
Under a physical delivery PPA, the purchaser, in this case the ammonia facility, would take full ownership of the electrons and renewable energy credits from the renewable energy project. The corporate buyer is responsible for monetizing the electrons in the wholesale electricity marketplace and/or via a product sale, such as green ammonia. Additionally, the corporate buyer may be responsible for any transmission costs associated with the renewable energy project.74 This arrangement matches the West Coast LCFS markets’ behind-the-meter requirements for process energy.
For example, the University of Minnesota’s West Central Research and Outreach Center near Morris recently announced plans and funding for a direct power delivery green ammonia project.75 The proposed project will build and operate a commercial-scale wind energy to green ammonia facility that produces one metric ton of low- and zero-carbon ammonia daily. The wind turbines powering the green ammonia facility will be located behind the meter and dedicate renewable energy credits (RECs) to green hydrogen and ammonia production.
Other projects could also be designed for this behind-the-meter set-up with siting of a facility to directly serve the plant. One could look at ammonia sales by county and compare the wind resource to identify areas of alignment, as depicted in Figure 10.76 Green ammonia facilities and associated renewable energy generation could also be sited close to existing ammonia storage facilities.
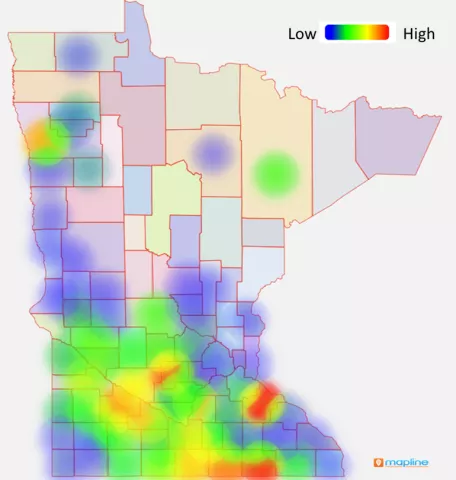
Figure 10: Match between wind potential and ammonia demand
As another example in Washington, the Douglas County Public Utilities District recently broke ground on a 5MW green hydrogen production facility based on their Wells Hydroelectric Project.77 The project puts to use a seasonal over-supply of hydroelectric power that previously resulted in negative power-pricing, much like occasional oversupply of wind in the Upper Midwest. The Douglas County PUD also holds a private contract with Toyota Motor North America to purchase the zero-carbon hydrogen output for fuel cell use.78 As a part of this development, a current bill in the Washington State Legislature would authorize and incentivize the expansion of hydrogen refueling stations via tax exemptions. As proposed, the bill would allow public utility districts and municipal utilities the ability to produce, use, sell, and distribute green electrolytic hydrogen.79
Minnesota policymakers could leverage a similar set of tax exemptions to encourage the construction of hydrogen fuel infrastructure and possibly enable a new revenue source for utilities that are transitioning to zero-carbon energy sources. This approach suggests aligning production with prime wind and solar resource locations, even if there are grid constraints, to maximize the renewable energy capacity of these facilities.
Virtual PPA Framework
Another option is the virtual power purchase agreement. Under a VPPA, the corporate buyer purchases power from the electric utility and the renewable energy certificates from the renewable energy project owner. The VPPA is entirely a financial transaction. The corporate buyer’s relationship with the utility remains unchanged at the retail electricity level. The corporate buyer still meets any energy needs through traditional utility energy supply, but it has “greened” its energy source through the purchase of renewable energy certificates which are measured and verified in megawatt-hour certificates by a third party.80 Virtual power purchase agreements for wind or solar energy in the Midwest might mirror the West Coast LCFS regulations regarding process energy by supplying power into the Midwest’s grid balancing authority, MISO. Arguably, this would give Midwestern-made clean fuels a competitive advantage in the fuels marketplace because of MISO’s larger footprint and lower-cost process energy.
Regulation that enables VPPAs for green ammonia would free up these facilities to site on the electric grid where they are most advantageous. This could include sections of the electric grid with congestion issues or near existing anhydrous ammonia storage facilities like those in Glenwood, Murdock, Garden City, or Rosemount mentioned above. Physical PPAs which would require sizing the renewable energy generation facility to a green ammonia plant size, and simultaneously anticipate the ammonia marketplace, could introduce capital risk and higher levelized costs.81 VPPAs for green ammonia would avoid this capital project complication.
However, whether green ammonia facilities could source renewables for green VPPAs in MISO requires more examination. A VPPA for renewable power could mitigate price uncertainty, but most of the wind-rich areas within MISO suffer from congestion and undergo economic curtailment at certain times of the year.82 Alternatively, a project could procure renewable energy certificates (RECs) on the open market. However, availability of RECs is from existing facilities is difficult to discern as the data from M-RETs public reports only show annual production and retirement data.83 Additionally, there is risk in relying on the market to procure RECs as prices can experience wide fluctuations.84 Availability of green power for VPPAs, or even through RECs, thus remains an open question.
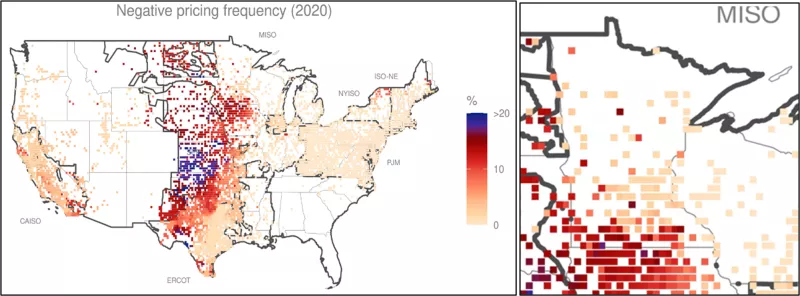
Figure 11: Berkeley Lab’s Renewables and Wholesale Electricity Prices Tool—Negative Price Frequency and detailed geographic location of areas where wind is economically curtailed because of lack of transmission capacity and wind energy volume.
Next: Conclusion